In exploring the action of microvesicles, Quan Lu and colleagues are mapping the next frontier of medical diagnostics and drug delivery
Surprises are expected in science. Large, double surprises are rare.
Earlier this year, Quan Lu and his colleagues published their discovery of a gene that could be a root cause of asthma. They found that a gene called gasdermin B—but not its neighboring genes, which had long been suspected of an asthma link—codes a protein that kills lung cells and may ultimately trigger asthma. For Lu, associate professor of environmental genetics and pathophysiology at the Harvard T.H. Chan School of Public Health, the finding was a breakthrough for a laboratory team that has long chased the molecular origins of asthma—a disease that afflicts nearly 27 million adults and children in the U.S. and kills more than 3,600 people a year.
But a chance follow-up by Lu’s team led to a thrilling second discovery. In rooting around for other genes that may be associated with asthma, the group came across a gene called ARRDC1 (for arrestin domain-containing 1). To their initial disappointment, Lu’s team did not find any direct role for the gene in asthma or in receptor signaling in the lung, as they had hypothesized. But they later discovered that the ARRDC1 protein has a unique capability: It can travel from inside to the surface of a cell to form tiny sacs, called microvesicles, which are subsequently released into the space around a cell. Through this singular mechanism, ARRDC1 protein can “bud” out of a cell, just like a virus.
The implications are dramatic. Microvesicles—tiny biological delivery trucks, the ubiquitous presence of which has been revealed only in the past decade—could generate new paradigms in basic biology, diagnostics, and treatment. In the not-too-distant future, their fleet may even be enlisted in novel ways to shut down tumors, track toxic exposures, or even turn off an asthma attack.
“This is a whole new form of intercellular communication. It’s going to change medicine,” says Philip Stahl, emeritus professor of cell biology at Washington University. “We are learning the way cells talk to each other, but we don’t know all the vocabulary yet. The heavy lifting in this field is still ahead of us.”
Microvesicles are part of a group of structures called extracellular vesicles, or EVs—the scientific study of which didn’t take off until 2006. The microvesicles that Lu’s team found are 100 nanometers in diameter—about 1/1,000th the width of a human hair and visible only at the highest magnification of electron microscopes. Yet these infinitesimal spheres may play a central and, until recently, completely unknown role in health and disease.
All cells make vesicles and push them through their cell membranes, where the structures sit, awaiting assignment. Once dispatched, they migrate in blood and other body fluids to target cells with the right molecular addresses, which will absorb them and unload their vital cargo—proteins, fats, and nucleic acids such as DNA and RNA—for basic biological functions such as coagulation and immune response. Scientists used to dismiss EVs as cellular debris—waste products of cellular activities. But they now believe these tiny floating itinerants are key mediators of cell communication.

The human body is like a cellular megacity, made up of some 37 trillion human cells and 100 trillion bacterial cells. To adapt to external stimuli, these cells have developed complex mechanisms of communication by which they can receive a message, transfer information across the plasma membrane, and launch changes within the cell in response to the message. In multicellular organisms, cells send and receive chemical messages constantly to coordinate the actions of distant organs, tissues, and cells.
As part of this process, every cell spins off 100 or more vesicles. According to Lu’s calculations, humans harbor about 5 to 10 quadrillion vesicles, each engaged in furious cell-to-cell signaling—a roar of conversations similar to that of a stock exchange floor. Today, scientists in the rapidly evolving science around vesicles are starting to listen in to these communications to fathom the secrets of both normal physiology and disease.
Most long-distance communication, or chemical signaling, between cells involves proteins or hormones that travel from one cell and bind, like a lock and key, to a new cell. The protein insulin, for example, is made in the pancreas and sent to muscle and fat cells, where the hormone helps cells absorb sugar. The ability of a cell to receive signals from other cells and then translate those signals into changes in cell behavior plays a crucial role in development and homeostasis. Conversely, the disruption of these intricately orchestrated events often causes the onset or progression of disease. Highly aggressive and advanced-stage forms of cancer, for example, generate more EVs than lower-grade forms of cancer.
Lu’s lab has been focusing on the vesicles that are generated by the budding of the ARRDC1 protein. He named these vesicles ARMMs (for ARRDC1-mediated microvesicles). He found that ARMMs contain NOTCH receptors—proteins involved in a wide range of critical physiological tasks, from embryonic development and tissue homeostasis to immunity and stem cell function. By delivering NOTCH cargoes from one cell to another cell at a distance, ARMMs set in motion a type of cell-to-cell communication based on NOTCH receptors and also carry signals from one tissue or one cell to another.
“This is a newly understood type of signaling,” Lu says. In classic hormone signaling, one protein or peptide is transferred in direct contact between cells. “But microvesicles such as ARMMs can carry many different kinds of molecules—not only proteins but also nucleic acids and lipids. This means that their signaling capacity is bigger than traditional hormones’. We used to think of microvesicle signaling as a message in a bottle, but the process is actually much more sophisticated and complicated than that analogy.”
“How do these vesicles know where to go? We need to understand the basic biology before we say we’re going to design this, engineer that. We want to learn how nature does it, then mimic that process and improve upon it.”
—Quan Lu, associate professor of environmental genetics and pathophysiology
Lu exudes a physical energy, frequently springing up from his chair to sketch out microvesicle architecture on an office whiteboard. Trim and wiry, he was an avid soccer player during his student years in China and has since taken up basketball, which he plays with his son on a backyard hoop. A faculty member at the Harvard Chan School for 10 years, he now leads a team of eight scientists in the emerging field of microvesicle research.
In Lu’s mind, the possible applications in therapeutics and public health are endless. In part, that’s because vesicles can travel to distant cells, where they are taken up and unpacked—extracting a gene, for example, that could combat cancer. Biologists could also insert molecules of their own choosing for direct delivery into cells. And because cells insert nucleic acids such as DNA and RNA from their own interiors into vesicles, the vesicles acquire a biological signature; vesicles from a tumor cell, for instance, will contain genes that identify their home as a tumor cell.
One of the most daunting problems in medicine is that human cells actively reject substances from the outside. To allow things in, a cell must open to its outside environment. But because extracellular vesicles are made by cells themselves, they are perceived by the body as “self” and serve as natural vehicles that can pass through cell barriers to deliver therapies, both artificial and natural, precisely where they are needed. Drugs that cannot be otherwise delivered inside cells could be loaded in a vesicle and delivered Trojan horse–like to their targets.
In this regard, Lu cites the p53 gene—“the most widely studied gene in medicine,” as he puts it, because it can shut down uncontrolled cell proliferation. Many tumors typically lack or have compromised p53, so they can grow unfettered. Lu and his colleagues are pursuing a strategy of adding p53 into vesicles to deliver it back into tumor cells and shut down their growth.
Lu holds two patents that bank on this advantageous biological capability of ARMMs. “In the patents, we proposed to swap molecules in ARMMs vesicles with therapeutic molecular cargoes,” he says. While clinical trials may be 10 years away, “the potential advantage and the scope of the application is huge, and the path forward is clear.” The possibilities include swapping in agents to reduce the lung inflammation triggered by diseases such as asthma or chronic obstructive pulmonary disease, or to deliver insulin to treat diabetes.
Lu is also refining methods to send drug-loaded vesicles to specific cells in the body, in the hopes of better targeting delivery, increasing effectiveness, and reducing toxicity. By injecting custom-made vesicles intravenously in animals, he intends to learn where the vesicles naturally move and how he might tweak the vesicles to point them toward specific body tissues. “In most therapeutic delivery platforms, therapeutics go to the liver, where detoxification happens. If we can show that our vesicles can go directly to tissues, bypassing the liver, that would be a big improvement.”
Vesicles can also be used as biomarkers to track the progress of diseases or environmental exposures. Studies already show that exposure to air pollution and lead affect the secretion of EVs. And because EVs are altered in disease states, it may be possible with a simple blood draw to measure the effects of air pollution—not just within individuals but across large populations as well.
A NEW PARADIGM FOR CELL COMMUNICATION
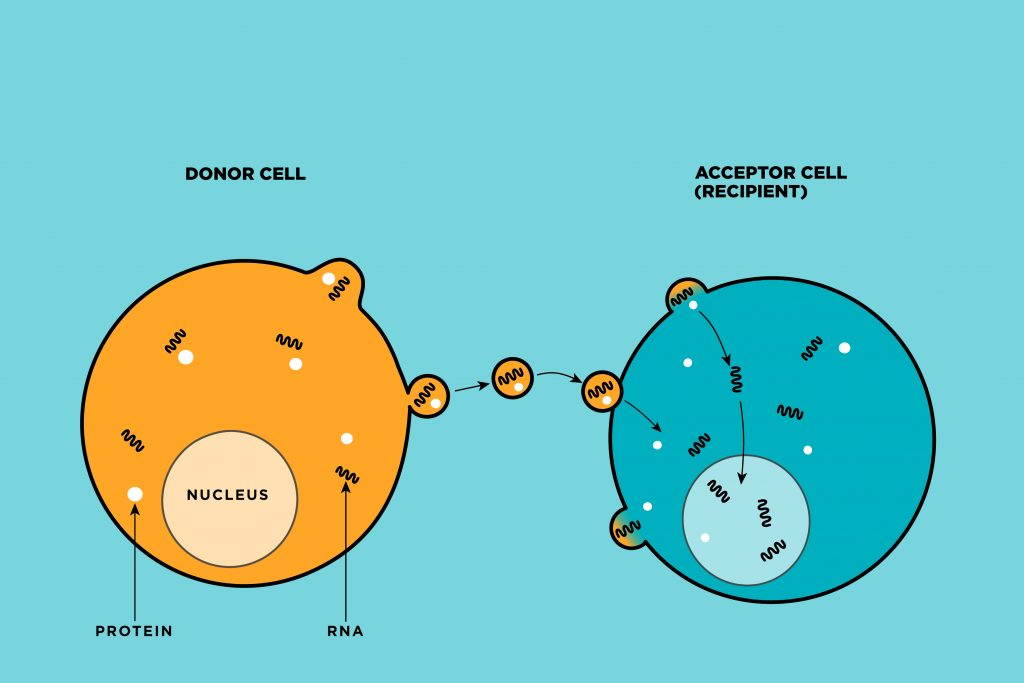
In the future, drawing on a simple blood or urine sample, a painless “liquid biopsy,” could conceivably spot a tumor by analyzing the contents of the vesicles shed by the tumor cell. Repeated sampling would allow researchers to track the changes in a tumor through changes in the vesicles.
Gökhan Hotamisligil, chair of the Department of Molecular Metabolism, where Lu has a secondary appointment, notes that, “Dr. Lu didn’t come to this school to study microvesicles. He was a technologist who brought genetic tools to public health issues. The greatest discoveries in health haven’t come from work intended to transform public health. Rather, they came from curious minds studying basic science.”
Quan Lu says he enjoys one great advantage in conducting this line of research in a school of public health: The bench researchers in his lab toil within a few corridors of physicians who treat patients in clinics—meaning the link to front-line medical practice is just a conversation away.
“We did not start out with the idea that we needed to deliver drugs or find biomarkers,” Lu says. “This project grew out of curiosity about the discovery that cells secrete these vesicles. We then asked: What are these structures? What can they do? What are their potential applications?”
The fundamental question, he adds, is “How do these vesicles know where to go? We need to understand the basic biology before we say we’re going to design this, engineer that. We want to learn how nature does it, then mimic that process and improve upon it.” Someday, Lu predicts, vesicles may be used to treat all human diseases. “I would like to be part of that.”
Philip J. Hilts is a Boston-based science writer and author, and former director of the Knight Science Journalism Fellowship at the Massachusetts Institute of Technology.
Photos: Kent Dayton
Illustration: Anne Hubbard